Laboratory of Exploration and Applied Geophysics
- Home
- Instruments and Personnel
- Methods and Applications
- Project and Scientific Interests
- Pubblications
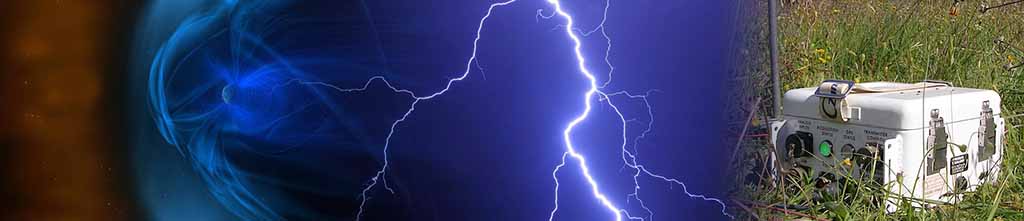
The science of geophysics applies the principles of physics to the study of the Earth. Exploration and applied geophysics exploit quantitative methods to image the Earth’s interior and its physical properties. Measurements at the Earth’s surface can reveal how the physical properties of the Earth’s interior vary in space (and possibly in time). Geophysics has to do with all aspects of the physics of the Earth and therefore is an essential support for the general needs of society.
The Laboratory of Exploration and Applied Geophysics at IGG focuses the research activities on the exploration of earth resources, mainly but not limited to geothermal resources and groundwater. Its researchers have been working since 1990 in the exploration of complex tectonic areas for crustal, tectonic, volcanological and geothermal investigations.
The Laboratory has acquisition capabilities of electromagnetic and geoelectric measurements exploiting the state-of-the-art with its recently renewed geophysical instrumentations. In addition, the Laboratory uses the most updated tools for the processing, analysis and inversion of geophysical data.
Research activities are ongoing to develop probabilistic and computational intelligence-based algorithms for solving the geophysical inverse problem, also jointly and/or constrained by external geological information.
Research activities are also focused on the integration of different sources of data (well, geological, geophysical) in 3D to unravel the complexity of the subsurface
INSTRUMENTS
The Laboratory of Exploration and Applied Geophysics was recently renewed and expanded with newly purchased instruments. The Lab is equipped with state-of-the-art instruments for the acquisition of magnetotelluric and geoelectric data:
Magnetotelluric (MT) broad-band equipment (0.0001 Hz – 1000 Hz):
Zonge system. High-resolution, multi-channel 32-bit systems for recording two electric field components (Ex, Ey) and three magnetic components (Hx, Hy, Hz). MT and AMT data can be acquired for imaging the subsurface resistivity.
Components:
- 3 ZEN HighRes receiver broad-band (0.0001 Hz a 1000 Hz). The receiver may acquire 6 synchronized channels at different sampling frequencies from 256 Hz to 4096 Hz. Each channel has mass storage of 8 GB, allowing continuous recording for various days.
- 8 magnetic antennas ANT/4 of ZONGE.
- Sets of non-polarizable electrodes of various types: Pb-PbCl (Wolf) and Ag/AgCl (Stelth)
- Miscellaneous accessories
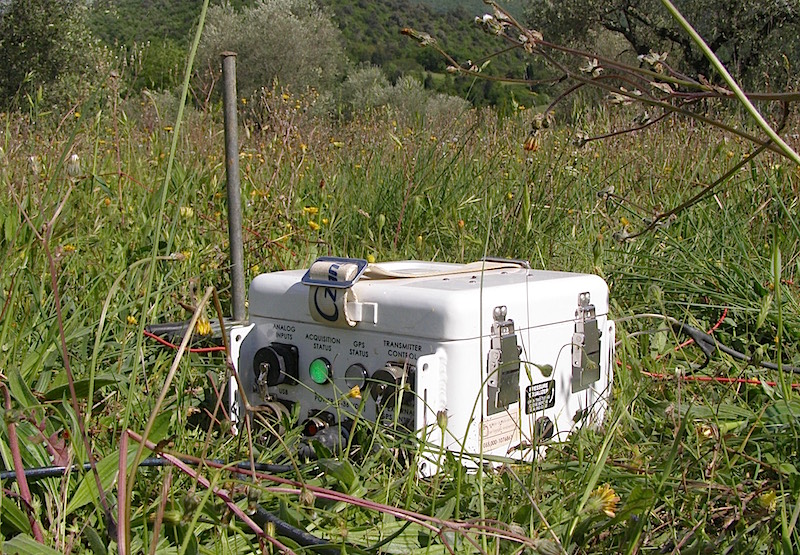
ABEM system. A standalone solution, multi-channel system for high power current transmission and accurate electrical potential measurements for resistivity/IP surveying:
Components:
- 1 Terrameter LS 2, 10 channels (up to 12), 48 electrodes (up to 81). Maximum output voltage 600 V, maximum output current 2500 mA, maximum output power 250 W.
- 2 multipole cables 24 takeouts each and 10 m spacing (480 m total length)
- Set of steel electrodes
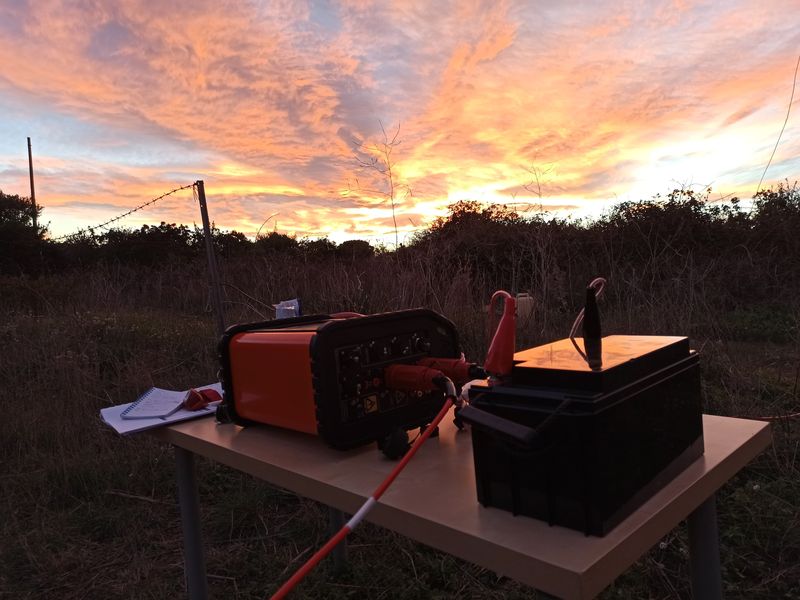
The Laboratory is also equipped with very old instruments, some of them stored in the Lab’s historical corner (such as the old V5 Phoenix equipment), whereas other instruments will be next assessed for a possible recovery:
Hybrid Natural and Controlled-Source Audio Magnetotelluric (CSAMT and AMT) equipment (0.1 Hz - 100 kHz):
Stratagem system by Geometrics. Stratagem’s hybrid-source technique exploits a hybrid source of signal from a combination of natural MT signals and man-made transmitter signals for the imaging of the subsurface resistivity
- 1 transmitter STRATAGEM with vertical loop antennas.
- 1 STRATAGEM receiver
- magnetic sensors
Magnetotelluric (MT) low-frequency equipment (0.0001 Hz - 0.03 Hz, 24 bit digital sampling):
2 NIMS (Narod Intelligent Magnetotelluric Systems) Receivers.
3 magnetometers (full 3-components fluxgate)
Gravity measurements:
1 Gravimeter
Equipment for data analysis and modelling:
Laptops for in-situ MT data processing
Desktop computer for high-performance data processing, editing, modelling
Access to HPC by CNR-IGG
Software
3 licences for Winglink (Schlumberger) for data processing, editing, 1D/2D modelling and 3D forward modelling of geophysical data
1 licence for Petrel (Schlumberger) for 3D geological modelling and data integration (managed by CNR-IGAG)
1 licence of Zonge software for data processing, editing, 1D and 2D modelling
1 licence of MODEM for 3D inversion of magnetotelluric data
Various open-source software for processing and inversion of geophysical data
In-house software developed by our Lab’s researchers for processing, editing, analyzing and inverting (also jointly) geophysical data. Computational Intelligent-based algorithms are the focus of such software
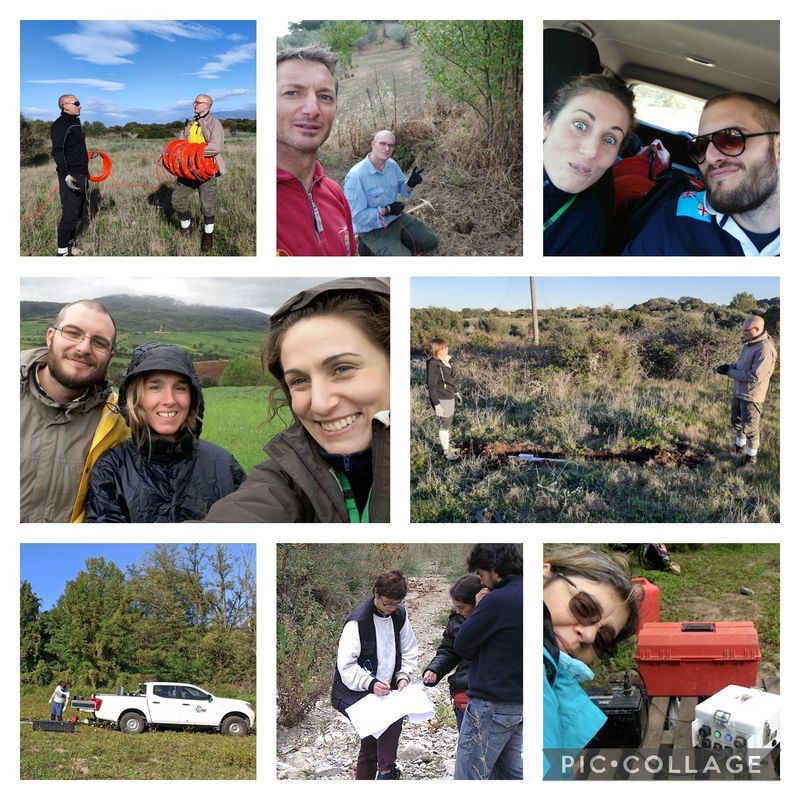
STAFF
Dr. Alessandro Santilano (Head of the Laboratory - CNR Researcher)
Dr. Adele Manzella (CNR Senior Researcher)
Dr. Serena Botteghi (CNR Researcher)
Dr. Assunta Donato (CNR Researcher)
CONTACTS
Phone:
050 6213417 (Laboratory)
050 6212571 (Dr. Alessandro Santilano)
050 6212392 (Dr. Adele Manzella)
050 6212387 (Dr. Serena Botteghi)
E-mail:
alessandro.santilano(at)igg.cnr(dot)it
adele.manzella(at)igg.cnr(dot)it
serena.botteghi(at)igg.cnr(dot)it
assunta.donato(at)igg.cnr(dot)it
METHODS
MAGNETOTELLURICS
Magnetotellurics (MT) is a geophysical electromagnetic passive method for determining the subsurface electrical resistivity by measuring the natural magnetic and electrical fields on the Earth’s surface with frequencies ranging between 10^4 and 10^-5 Hz. The energy source corresponds to the natural time-varying electromagnetic waves generated in the atmosphere and magnetosphere by different physical processes such as: i) Schumann resonance of lightning discharges, for higher frequencies fields, and the interaction between the Earth’s magnetosphere and the solar wind plasma, for lower frequencies fields.
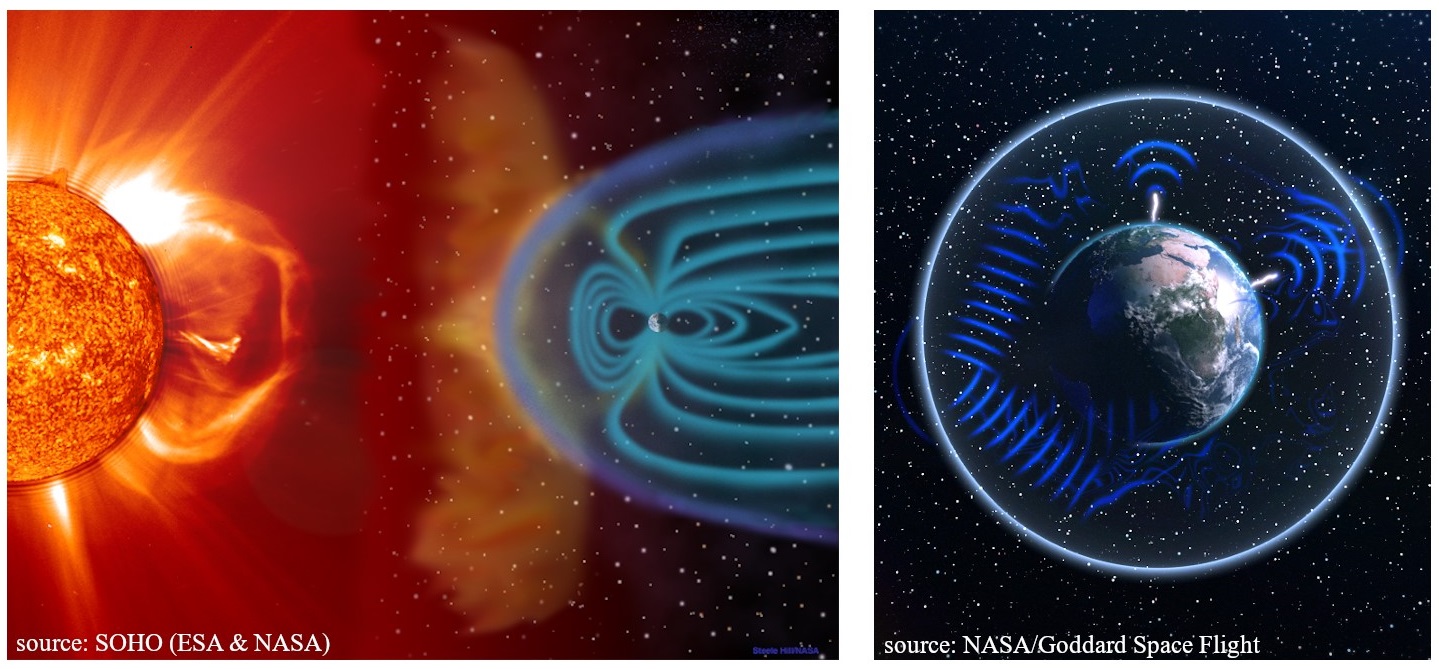
Radio-magnetotellurics (RMT), Audio-Magnetotellurics (AMT), Broad-Band Magnetotellurics (BBMT) and Long-Period Magnetotellurics (LMT) differ only for the measured frequencies. Being the method based on electromagnetic induction and diffusion processes, the investigated depth depends upon the measured frequencies (and the subsurface resistivity) according to the skin effect. The higher the frequencies, the shallower the investigation depth. This method is suitable for imaging the resistivity from tens of meters to hundreds of kilometres. AMT has a shallow investigation depth, usually limited to 1-3 km.
Controlled Source Magnetotelluric (CSMT) uses the same theoretical base of MT and an artificial signal transmitter, and is used to prevent low signal-to-noise ratios where cultural noise and a weak natural signal can be present.
Simultaneous measurements of orthogonal components of the electric and magnetic fields at the surface provide data for computing the transfer function (impedance) as a function of frequency. Impedance, in turn, is used to construct earth resistivity models.
Five synchronized measurements are recorded at each MT site: the electric field in two horizontal directions, the magnetic field in the two horizontal and the vertical directions, the horizontal directions being orthogonal. A typical MT station for data acquisition consists of two pairs of electrodes set up as orthogonal dipoles with a length between 50 and 100 m, and three magnetometers also set up in orthogonal (two horizontal and vertical) directions.
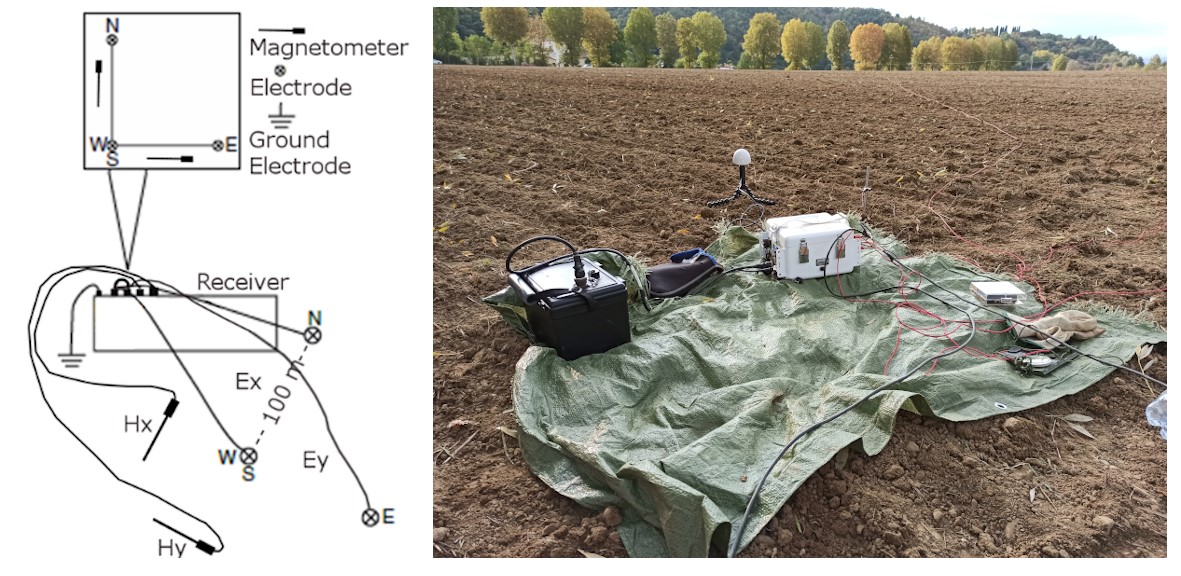
The magnetotelluric method has not environmental impacts either visually since sensors are located in holes and little trenches. The survey area is explored using several sites, whose location is chosen to try to cover the area uniformly. Care is also taken to avoid noisy, difficult access and rough topography sites.
Among the main application fields of the MT prospection method, CNR-IGG has worked in particular in:
- Geothermal exploration to investigate fluid pathways and heat sources of geothermal resources
- Volcanological exploration, to investigate the depth and volumes of magmatic chambers and volcanic edifices in active Italian volcanoes (Vesuvius and Etna)
- Hydrogeological exploration
- Deep crustal exploration
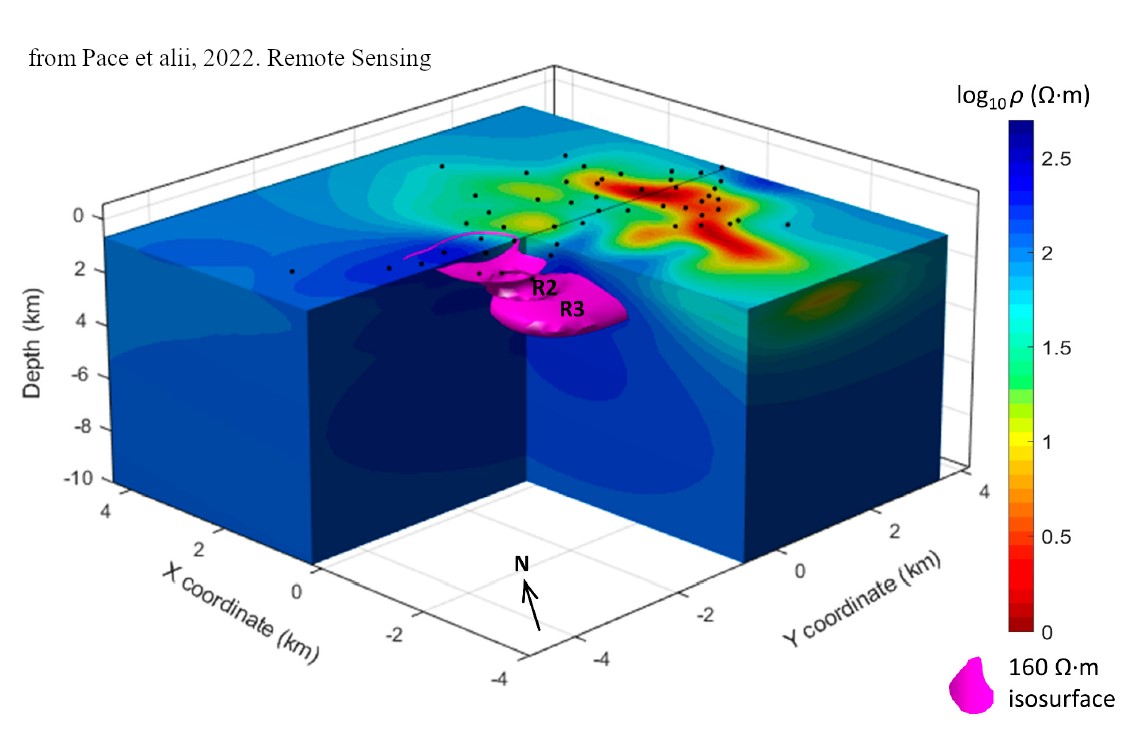
GEOELECTRIC
The geoelectric is one of the oldest and most commonly used geophysical exploration methods. The first pioneering applications were made almost contemporary by Conrad Schlumberger and Frank Wenner at the beginning of the 1900’s. The method is widely adopted for earth resources exploration, environmental and civil engineering, and archaeological investigations. Since its first use till the late 1980's, it has been used essentially as a one-dimensional (1-D) mapping method, progressively replaced by multi-channel and multi-electrode acquisition systems and multidimensional algorithms for modelling and inversion. State-of-the-art systems allow data acquisition with automatic protocols to retrieve 2D or 3D tomographic reconstruction. This technique is called electric resistivity tomography (ERT).
The theory is based on Ohm's law to relate the (unknown) electrical resistivity to current and electrical potential.
A typical acquisition implies the injection of a current into the ground through two current electrodes AB (usually metal stakes) and measuring the difference in the resulting voltage at two potential electrodes MN.
Through internal (programmable) switching circuitry, multi-electrode resistivity meters, automatically select the appropriate 4 electrodes for each measurement. Hence, any array configuration can be used. By making measurements with different spacing at different locations, a subsurface 2-D profile (or 3D model) is obtained.
As a rule of thumb, the depth of investigation is about 1/5 to 1/6 of the layout length.
Among the main application fields of the geoelectric prospection method, CNR-IGG has worked in particular in:
- Geothermal exploration to investigate fluid pathways
- Hydrogeological studies (coming soon also a survey in Artic environment)
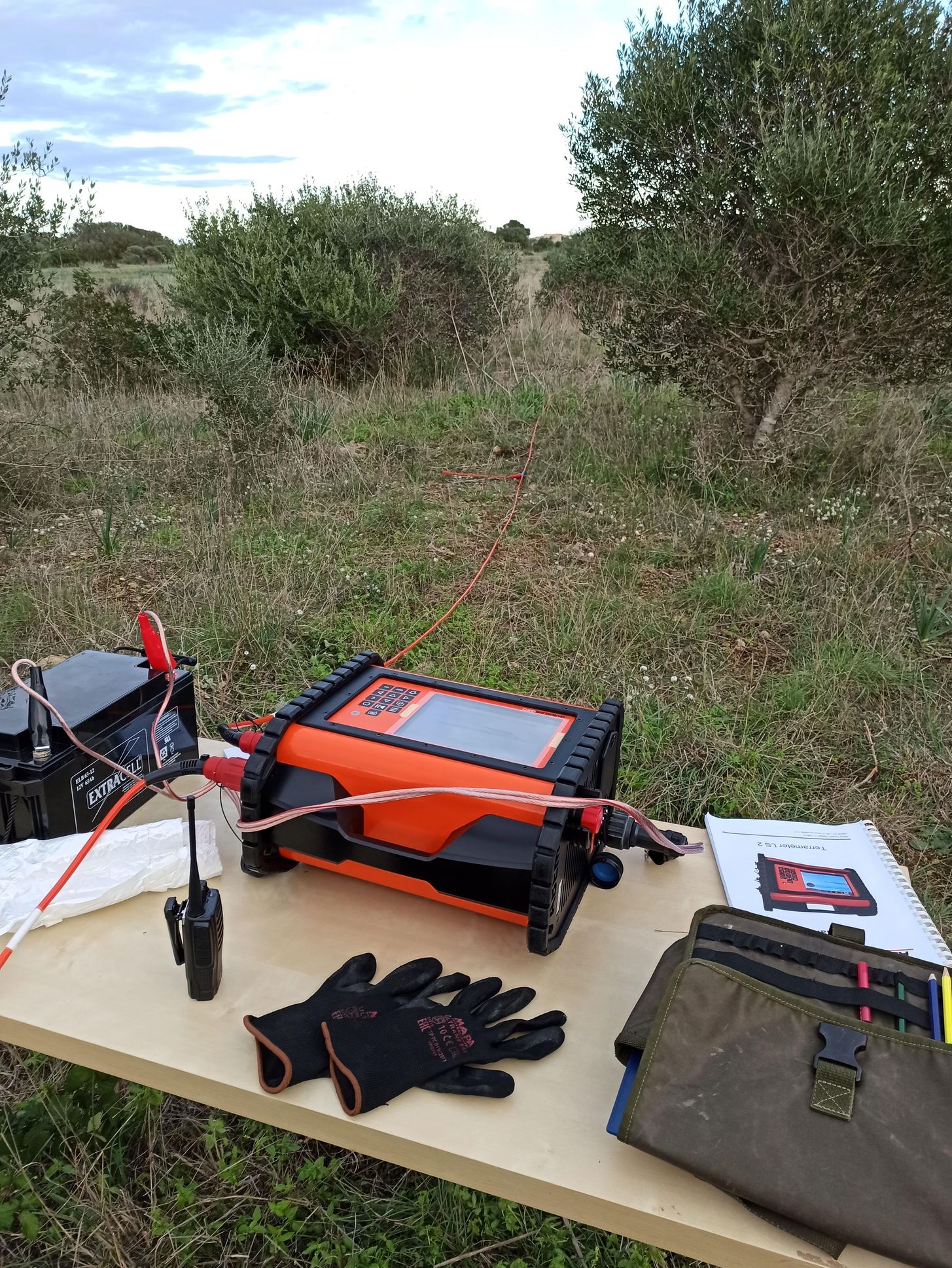
INVERSE MODELLING
In geophysics, a complete understanding of the physics behind the methods (forward operator) allows the measured data to be related to the model parameters of the earth. The inverse problem is to find the model parameters “m”, given certain observed data “d”. By solving the inverse problem, the indirect esteem of the electrical resistivity of the subsoil is retrieved from the analysis of the data measured at the surface (magnetotelluric, geoelectric, etc.). The problem in magnetotelluric is
ill-posed due to its instability and can demand very high computational resources. The methods for solving the inverse problem can be classified into deterministic and probabilistic methods. Deterministic methods are the conventional means of estimating the resistivity models.
The MT response is particularly affected by the dimensionality of the measured impedance tensor. The literature is rich in deterministic schemes for 1D, 2D, and 3D inversions, with 3D inversion being the main focus of current scientific research. The model parameters are generally solved iteratively by minimizing an objective function operator and exploiting differential operators.
The procedure may reach a local minimum, also depending on how close the starting model is to the global minimum.
In the Exploration and Applied Geophysics Lab, we exploit state-of-the-art codes for the inversion of MT and geoelectric data in 1D-2D-3D.
Conversely, the probabilistic approach is less conventional and is still a matter of research. The probabilistic methods do not imply any differential operator, but the model space is sampled randomly or according to some strategy.
Many earth models are proposed in the probabilistic methods, and the theoretical data are compared with the observed data. Then, the minimization function is directly estimated to retrieve the best model. The philosophy of the probabilistic approach, which can be considered an optimization procedure, is to explore a broader space solution to seek a global solution to the problem.
In the Exploration and Applied Geophysics Lab, in collaboration with other partners, we develop Computational-Intelligence based algorithms to solve the geophysical inverse problem also jointly.
PROJECTS
The Laboratory of Exploration and Applied Geophysics was involved in various scientific projects and collaborated with many universities and research centres at the national and international levels.
Recent scientific projects:
- ICEtoFLUX (Arctic Research Programme “PRA”, 2022-2024): “HydrologIcal changes in ArctiC Environments and water-driven biogeochemical FLUXes”
- GECO (EU-H2020 project, 2018-2022): “Geothermal Emission Control”
- GEMEX (EU-H2020 Project, 2016-2020): “Cooperation in Geothermal energy research Europe-Mexico for development of Enhanced Geothermal Systems and Superhot Geothermal Systems”
- IMAGE (EU-FP7 Project, 2013-2017): "Integrated Methods for Advanced Geothermal Exploration”
- “Geothermal Atlas of Southern Italy” (CNR per il Mezzogiorno Project, 2011-2016)
- VIGOR (POI-FESR Project, 2010-2014): “Geothermal potential assessment of Convergence Regions”
- I-GET (EU-FP6 Project, 2005-2009): “Integrated Geophysical Exploration Technologies”
- Si.Ri.Pro (PON Project 2004-2010): "SIsmica a RIflessione PROfonda, Metodologie integrate per l'esplorazione geofisica avanzata lungo un transetto crostale, Sicilia (Deep Reflection Seismic, Integrated methodologies for advanced geophysical exploration along a crustal transect in Sicily)”
- INTAS EU Project (2004-2006): "Three-dimension electromagnetic and thermal tomography of the active crustal zones"
Scientific collaborations and applications (with or without field activity):
- Geothermal investigation in Italy; Slovakia; Iceland; Ethiopia; Mexico; Tibet; Australia; Sri Lanka
- Hydrogeological exploration: Svalbard (Artic, coming soon); Pianosa Island; Equi Terme; Monte Amiata (Italy)
- Support for environmental impact assessment for the Medici’s estate of Cafaggiolo by magnetotellurics (Barberino di Mugello, FI)
- Crustal investigation: MT along some profiles of the CROP Project (Italy); Czech Republic; Antarctic
- Volcanological exploration in INGV projects (Vesuvio, Etna)
SCIENTIFIC INTEREST
- Geothermal, crustal and volcanological exploration
- Development of computational intelligence-based algorithms for geophysical modelling
- Integrated modelling for earth resources exploration
SELECTED PUBLICATIONS
Pace, F., Marti, A., Queralt, P., Santilano, A., Manzella, A., Ledo, J., Godio, A., 2022. Three-dimensional magnetotelluric characterization of the Travale geothermal field (Italy). Remote Sens. 2022, 14(3), 542
Santilano, A., Godio, A., Manzella, A. 2018. Particle swarm optimization for simultaneous analysis of magnetotelluric and time-domain electromagnetic data. Geophysics, 83 (3), E151-E159
Godio, A. and Santilano, A. 2018. On the optimization of electromagnetic geophysical data: application of the PSO algorithm. Journal of Applied Geophysics 148, 163-174
Santilano, A., Donato, A., Galgaro, A., Montanari, D., Menghini, A., Viezzoli, A., Di Sipio, E., Destro, E., Manzella, A. 2016. An integrated 3D approach to assess the geothermal heat-exchange potential: the case study of western Sicily (southern Italy). Renewable Energy 97 (2016) 611-624
Santilano, A., Manzella, A., Gianelli, G., Donato, A., Gola, G., Nardini, I., Trumpy, E., Botteghi, S., 2015. Convective, Intrusive Geothermal Plays: what about tectonics? Geothermal energy Science, 3, 51-59
Molli, G., Doveri, M., Manzella, A., Bonini, L., Botti, F., Menichini, M., Montanari, D., Trumpy, E., Ungari, A., Vaselli, L., 2015 Surface-subsurface structural architecture and groundwater flow of the Equi Terme hydrothermal area, northern Tuscany Italy. Ital. J. Geosci., Vol. 134, No. 3, pp. 442-457
Spichak V. and Manzella A., 2009. Electromagnetic sounding of geothermal zones. Journal of Applied Geophysics, 68, 459-478
Armadillo E., Bozzo E., Caneva G., Manzella A., Ranieri G. 2007. Imaging deep and shallow structures by electromagnetic soundings moving from the Transantarctic Mountains to the Wilkes Subglacial Basin. Terra Antartica Reports. Issue 13, Pages 65-74
Manzella, A., and Zaja, A. 2006: Volcanic structure of the southern sector of Mt. Etna after the 2001 and 2002 eruptions defined by magnetotelluric measurements. Bulletin of Volcanology 69 (1), 41-50
Oskooi, B., Pedersen, L.B., Smirnov, M., Arnason, K., Eysteinsson, H., Manzella, A., the DGP Working Group 2005. The deep geothermal structure of the Mid-Atlantic Ridge deduced from MT data in SW Iceland. Phys. Earth Planet. Int., 150, 183-195
Manzella, A., 2004. Resistivity and heterogeneity of Earth crust in an active tectonic region, southern Tuscany, Italy. Annals of Geophysics, 47, 107-118
Manzella, A., Volpi, G., Zaja, A., Meju, M. 2004. Combined TEM-MT investigation of shallow-depth resistivity structure of Mt. Somma-Vesuvius. J. Volc. and Geoth. Res., 131, 19-32
Volpi, G., Manzella, A., Fiordelisi, A. 2003. Investigation of geothermal structures by magnetotellurics (MT): an example from the Mt. Amiata area, Italy. Geothermics, 32, 131-145
Di Mauro D., G. Volpi , A. Manzella, A. Zaja, N. Praticelli, V. Cerv, J. Pek De Santis, A., 1999. Magnetotelluric investigations of the seismically active region of northern bohemia: preliminary results. Annali di Geofisica, 42, n.1, 39-48, 1999
J. C. Larsen, R. L. Mackie, A. Manzella, A. Fiordelisi, Rieven, S. 1996. Robust smooth magnetotelluric transfer functions. Geophys. J. Int., 124, Pag. 801-819.